Research
We focus on researching novel quantum technology like narrow-band sources of entanglement, long-distance quantum networks and quantum sensing, as well as on fundamental questions of physics – can there be quantum superposition of gravitational fields, do we need to quantize gravity? Can we entangle objects via their relative gravitational interaction? Some of these questions may have to be addressed in a space environment. To this end, we are working on proof-of-principle experiments on ground and on technology development for future space missions.
Entanglement and quantum communication
- Sources of Entanglement
Entanglement will be a key resource for future quantum networks. For example, it allows the teleportation of quantum states over arbitrary distances, enabling distributed quantum computing and quantum sensing and overcoming the losses in optical fiber networks by implementing quantum repeaters. To cover global distances, it will be imperative to realize such quantum repeaters. These typically rely on the teleportation (or “swapping”) of entanglement between distant nodes and its efficient storage in quantum memories. To enable this core requirement of future quantum networks, our group has been developing novel types of entanglement sources using cavity-enhanced nonlinear optical processes. - Distributing Entanglement
Optical fiber connections are near ubiquitous nowadays due to major, long-term investments by many governments. No not duplicate these efforts for future quantum networks, it makes sense to use that existing infrastructure to distribute entangled photons. This requires the photons to have a compatible wavelength (typically “o-band” or “c-band”), and it has to be possible to separate the single photons used or quantum communication from strong classical (not quantum) background in the same or nearby fibers. Because most classical background is in the c-band, we are implementing sources in the o-band to facilitate separating quantum from classical communication signals.
Sometimes, a fiber connection is not possible between two points. In this case, sending entangled photons via free-space optical links is an attractive alternative. Especially for global distances, free-space links using optical ground stations and satellites is very attractive. We are working on establishing test links between our faculty and the Jozef Stefan Institute to demonstrate the distribution and the storage of narrowband entanglement in atomic quantum memories.
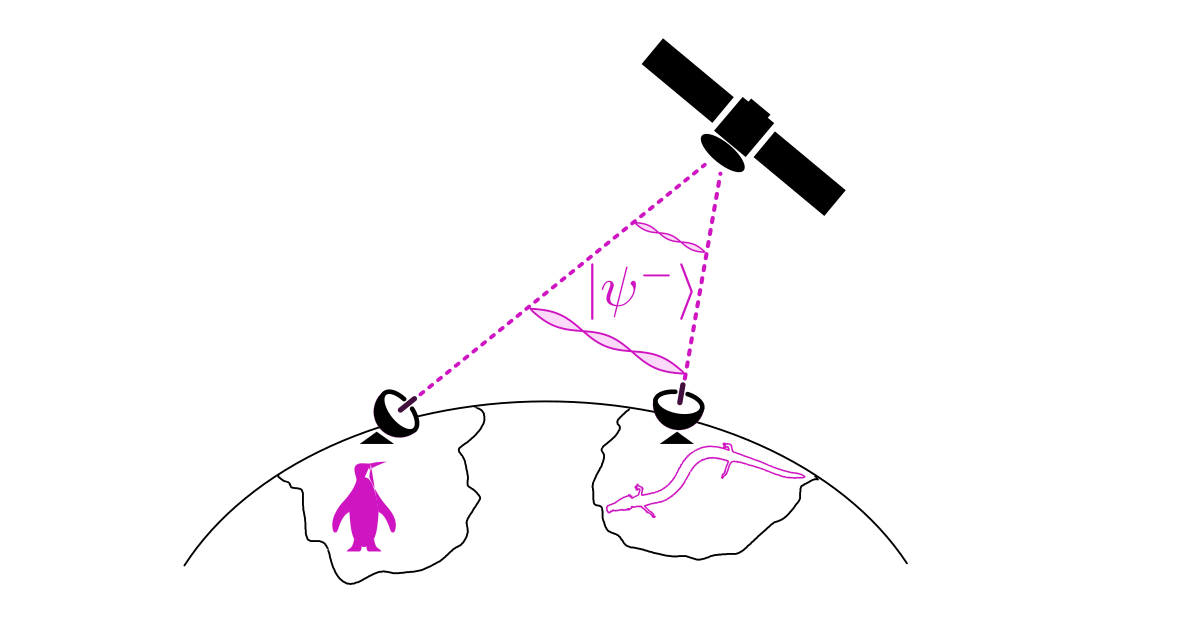
- National and International Quantum Networks
Since 2023, we have been a key member of the SiQUID project to implement a Slovene quantum communication infrastructure. In this context, we have been working on the implementation of demonstrators for state-of-the-art sources of entanglement, industrialized sources of entanglement, and of high-bandwidth lab demonstrators of prepare-and-measure quantum cryptography.
Quantum optomechanics with optically trapped particles
Optically trapping dielectric particles (sub-micron to micron size) in ultra-high vacuum in principle allows to control the motion of the trapped particles on the quantum level. Such optomechanical systems promise allowing to isolate the motion of the particles very well from coupling to the environment, e.g., via vibrations. Very requantum networks. For example, it allowscently, several groups have successfully demonstrated cooling the motion of such trapped particles close to the quantum ground state of motion, and researchers are now exploring ways of preparing the center-of-mass motion as well as rotational degrees of freedom of optically trapped particles in non-classical states. Such system promise high-precision sensors, the implementation of quantum transducers, where the optomechanical systems can, e.g., couple to optical fields but also to magnetic fields or microwave fields, and potentially to use optomechanical systems as quantum memories for storing quantum information. Moreover, quantum optomechanics with optically trapped particles are a very promising platform for testing the foundations of quantum physics (see below).
In our lab, we are currently working towards tackling several key challenges we need to face before we can harness the full potential of this architecture. Some examples are: (1) how can we reliably and on demand load individual sub-micron test particles into an optical trap in ultra-high vacuum and, potentially, at cryogenic temperatures? (2) how can we guide and trap such particles, and how can we ensure that they are not charged (charges could introduce excessive coupling to the environment)? (3) How can we keep the particles at low temperatures despite interacting with them via optical fields? In our research group, we currently address the first two points in a research project, where we aim to first optically trap test particles at low vacuum and then to guide them through a hollow-core photonic-crystal fiber into a ultra-high-vacuum chamber. You can find more details on the project page.
Testing the foundations of quantum physics
Quantum optomechanics with optically trapped particles promises excellent decoupling between the center-of-mass motion of the optomechanical system and the environment. Moreover, one can release optically trapped particles from the trapping potential to study the free quantum evolution of test particles to study the evolution of macroscopic superpositions and matter-wave interferometry with massive test particles. As we go to higher-mass test particles, we may eventually see deviations from the predictions of quantum physics as gravitational effects become more significant. As we probe parameter regimes where quantum physics has not been tested yet, such experiments also have the potential to allow testing for deviations from the standard model of particle physics, e.g., due to coupling to exotic matter or dark matter.
In our lab, we aim to develop novel ways to prepare macroscopic superpositions and to test the free quantum evolution of massive test particles. Eventually, we plan to perform such experiments in microgravity, in orbit, and in a deep-space fundamental science mission (the MAQRO proposal – see below).
Experiments in microgravity and in space – towards the MAQRO space mission
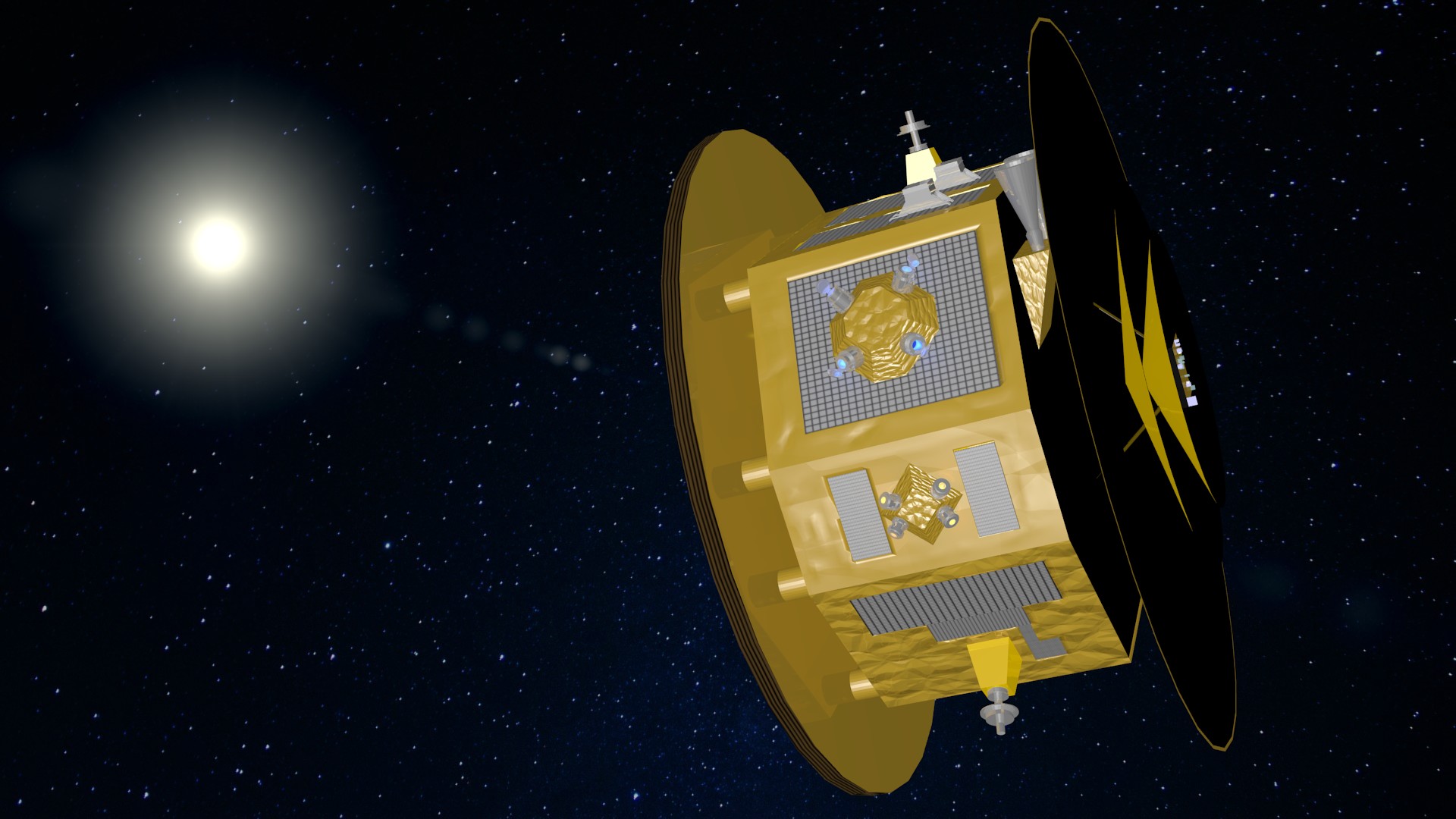
In 2010, R. Kaltenbaek and others proposed the fundamental-science space mission MAQRO, which would use quantum optomechanics and matter-wave interferometry with massive dielectric test particles to test the predictions of quantum physics with test masses orders of magnitude beyond what was then considered to be possible in Earth-based experiments. Over the years, the mission proposal was investigated in increasing detail, and the concept formed the basis for an in-depth feasibility study by the European Space Agency (ESA) in 2018. This “QPPF” study concluded that the mission concept is feasible in principle, but that we will have to increase the technological readiness of key components, and that we will have to address three critical issues (achieving the necessary vacuum and shielding from radiation in space, implementing a reliable loading and guiding mechanism for the test particles, demonstrating a reliable way for preparing the macroscopic quantum superpositions without introducing excessive decoherence). In order to tackle these challenges, we submitted a white paper for a NASA research campaign in 2021, and we are collaborating with other research teams to develop technology, proof-of-principle experiments on ground and in micro-gravity, and we plan to implement in-orbit demonstrators within the next ten years.